Powered by PacBio: Selected publications from April 2024
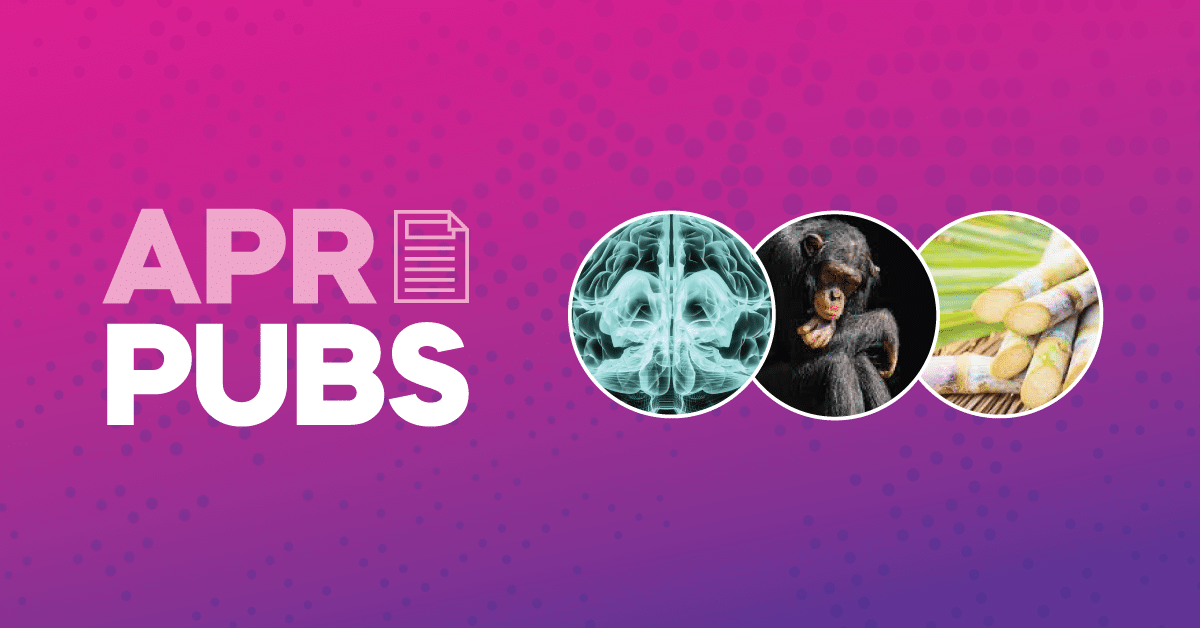
PacBio HiFi sequencing technology continues to be the tool of choice for genomics professionals working at the forefront of discovery, enabling them to pursue new avenues of exploration across diverse…